An FAA Type Certificate is issued to signify an aircraft is manufactured according to an approved design, and that the design ensures compliance with airworthiness requirements. 14 CFR Part 27-Normal Category Rotorcraft specifies these design and airworthiness requirements for rotorcraft weighing 7000 lbs or less or nine passenger seats or less. 14 CFR Part 29-Transport Category Rotorcraft would apply to rotorcraft over 7000 lbs and 10 or more passenger seats.
However, let me begin with a little historical perspective on helicopter certification standards. Originally, aircraft airworthiness standards used between 1926 and 1938 were called Aeronautical Bulletins. In 1934 Aeronautical Bulletin 7a established three classes of aircraft; normal airplanes, light airplanes (less than 1000 lbs) and gliders along with the airworthiness standards each class had to meet. Not surprisingly, rotorcraft were not yet included. In 1938 the Civil Aeronautics Authority (CAA) established the Civil Air Regulations (CAR) and in May 1946 created CAR 6, setting the certification basis for early rotorcraft designs. CAR 6 and its later amendments established the airworthiness standards for all helicopters certified in the 1940s, 50s and 60s.

In 1965 CAR 6 was replaced by new, tougher standards contained in 14 CFR Part 27. However, no helicopter was certified under Part 27 until the late 1970s (In 1979 the Robinson R22 became the first helicopter certificated under Part 27.) because of one giant loophole. This loophole states, in effect, that if the manufacturer makes a modification to a helicopter model (For example, adding a rotor blade.) this modification can often be approved under the rules in existence when the model received its original type certificate instead of the current rules. A good example of this is the difference between a Jet Ranger (BH206) and a Long Ranger (BH206L). They have a different rotor system, engine, drive train and fuselage. The only real similarity between the two is the model number, both are BH206s. By calling the Long Ranger a 206, rather than a 207 or a 208, Bell could go back to most of the certification standards in effect when the 206 received its type certificate in 1964 instead of FAR Part 27 that was in effect in 1975 when the Long Ranger was introduced. The FAA can include specific later requirements that will be detailed in the Type Certificate Date Sheet. McDonnell Douglas took advantage of the same loophole with the E model 500 (HU369E). Again, look at the differences between the 500C model and the 500E model; different number of rotor blades and “T” tail to name just a couple. But again, by calling the E model a HU369, McDonnell Douglas was able to certify it in 1982 under the standards that were in existence in 1964 when the 369 received its original type certificate. Manufactures do this because it’s typically cheaper and easier to use the older rules. I think it’s important to note that Robinson could have used this loophole with the R66 certification, thereby avoiding many new, time-consuming and expensive changes to Part 27, but choose not to. To a pilot this difference in certification standards is most clearly seen in the respective Rotorcraft Flight Manuals (RFM). Many items in the RFM or Pilot’s Operating Handbook (POH) are required by Part 27 that were not required by the CAR 6 rules; maximum glide distance and minimum rate of descent configurations are two examples. However, the FAA has recently adopted a “changed product rule” to help close this loophole by deciding for each modification which set of rules will apply. 14 CFR Part 27 has been amended several times since its creation to increase performance and safety standards.
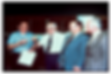

FAR Part 27 contains a number of areas in which satisfactory helicopter design is demonstrated by flight tests. Extensive tests are required for stress and strain analysis, controllability, stability, performance and development of the height-velocity diagram. Additional areas consist of cooling tests, ground endurance and function & reliability testing. Robinson uses 3000 ft density altitude for most of the in-flight testing. However, Part 27, unlike the old CAR 6 rules, also requires the manufacture to account for higher density altitudes. For example, a height -velocity diagram and hover controllability must be actually demonstrated to 7000 ft density altitude whereas the old rules did not require any high-altitude testing. During the flight tests the aircraft is operated under an experimental airworthiness certificate for research and development.
STRESS/STRAIN ANALYSIS
Stress is defined as an object’s internal resisting force and is determined by the load on an object or part divided by the part’s cross-section area. Strain is the amount of deformation or mechanical movement that occurs when a force or load is placed on a part. The stress/strain portion of the testing is used to determine the service life or component times for all the critical components and is one the most time-consuming tests because of the large number of conditions that need to be tested and the extensive test instrumentation required. Two factors must be determined to define a part’s service life. First, how much load a part is exposed to during flight and second, how much load a part can undergo until it fails. The in-flight load is determined by an in-flight strain survey. A strain gauge is a device used to measure the amount of deformation or strain at a specific place on a part. It is a small metallic foil pattern that converts the mechanical movement into an electrical signal. As the strain on the object changes the electrical resistance in the foil pattern also changes. When these strain gauges are connected in an array called a Wheatstone bridge (not to be confused by the rock band of the same name, really!) the change in resistance and the amount of strain can be measured. The strain gauges are very small, approximately 5mm square and are placed at numerous locations on one of the helicopter’s components, such as a rotor blade (see below).
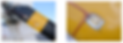
The aircraft is then flown in hundreds of different flight conditions i.e. at different bank angles, airspeeds, weights, power settings, centers of gravity, in cruise, climbs, descents, power on and power off recording the strain on, in this example, the rotor blade. Once the strain survey is completed, the engineers know the amount of strain or load the blade encounters during flight. Next the amount of load or length of time at a given load the blade can endure until failure is determined. This is done during a ground test in a machine designed to duplicate the highest in-flight loads on the blade. The machine is run day and night until the part fails. Once the engineers know how much load the blade is exposed to and how much load the blade can withstand until failure the service life can be calculated. This strain survey is conducted on all critical components. The R22 has 15 life-limited components and both the R44 & R66 has 21 so this is, as I’ve said, a very time-consuming process. For more details please see the essay on “Tired Metal”.
CONTROLLABILITY TESTING
The helicopter must have enough control authority to prevent the flight controls from contacting a control stop under certain critical flight conditions. For example, in forward flight at maximum speed and aft center of gravity, the cyclic cannot contact the forward stop (Robinson actually uses ¾”, measured from the top of the cyclic to the control stop for this test.). Controllability testing is conducted at a number of critical weights, center of gravities and flight conditions at speeds up to and, in some cases, above Vne. Maneuvers include power on flight, steady state autorotations and throttle chops with a 1 second delay prior to lowering the collective. Hover controllability is tested at both sea level and at high density altitudes. Part 27 requires the helicopter to demonstrate hover controllability in winds from any direction up to 17 knots (20 mph) and up to at least 7000 feet density altitude. For these tests, first weight is attached to the left skid to load the helicopter to the left lateral CG limit. Using a pace car, the aircraft is hovered in right sideward flight at 17 knots. Next, the test is repeated at the right lateral CG limit and finally, the weight is put on the toes of the skids and rearward flight at the forward CG is tested. If the controls do not hit a control stop and the test pilot is satisfied with the basic feel, the helicopter is considered controllable at a hover. Then it’s up to 7000 ft density altitude to repeat the test. It should be noted that in the helicopter’s Pilot Operating Manual, Part 27 allows the manufacture to extrapolate 2000 feet above the density altitude this test is actually conducted at. So, when you see 9800 ft DA in the R22 POH but 9600 ft DA in the R44 Raven I POH, it is not a reflection of a different capability, rather the difference in the density altitude on the day the test was completed.
STABILITY TESTING
Stability testing involves loading the helicopter to critical weights and center of gravity (usually the aft CG) and verifying the controls move in an expected direction (i.e. forward cyclic to go faster, aft cyclic to go slower) This static longitudinal stability (fore and aft cyclic) is tested in a climb, cruise and autorotation. The dynamics of small helicopters can make this more difficult to accomplish than one might think, hence the variety of creative stabilizers we see on many small helicopters. Static directional stability (stability about the yaw axis) is tested in both climb and autorotation. Since the direction and amount of flight control movement is really what’s being tested here, instrumentation to precisely measure flight control positions is required.
PREFORMANCE TESTING
During this phase, in and out-of-ground effect hover testing will be accomplished to obtain the data for development of the IGE and OGE hover charts in the POH. IGE testing is done at a 2 foot hover while the OGE tests are conducted at 1.5 times the rotor diameter above the ground. Hover testing is done at sea level and at a high density altitude. As with the hover controllability tests, Part 27 allows the manufacture to extrapolate, in this case, 4000 feet above the density altitude at which the tests were actually conducted. A change to Part 27 which occurred after the R22 certification but before the R44 certification requires the engineers to calculate the effect of an 80% humidity condition into the hover performance charts. The interpretation of this requirement changed again for the Raven II so for a detailed explanation see the essay on “IGE/OGE Hover Performance”. Climb and glide performance information such as best rate of climb speed, maximum glide distance and minimum rate of descent for autorotation will also be determined in this phase of testing.
Essentially, the manufacture has three performance factors; gross weight, Vne and service life that can be juggled depending on desired goals. For example, a higher Vne could be achieved by reducing the gross weight or lowering component service lives. Or, higher component lives might be achieved by lowering the gross weight and/or the Vne. This can be seen in both the R44 and Bell 206. At the higher gross weights, the Vne in both helicopters is reduced. I think it’s fair to say that at Robinson certain performance goals are in mind and once those goals are reached with an acceptable margin of safety development testing is stopped to focus on production.
TYPE INSPECTION AUTHORIZATION
After the manufacturer has completed the stress analysis, controllability, stability, and performance tests and is satisfied with the results, a report containing all the company data is submitted to the FAA. After a thorough review, the FAA will issue a Type Inspection Authorization, or TIA. During the TIA testing the FAA will select critical areas from the company’s report and repeat the tests with an FAA test pilot.
THE HEIGHT–VELOCITY DIAGRAM
The Height-Velocity (H-V) Diagram is part of the TIA testing and, for obvious reasons, is typically the last serious flight test. The H-V diagram indicates the combinations of airspeed and height above the ground that will allow an average pilot to successfully complete a landing after an engine failure. A couple of interesting terms there; “average pilot” and “successfully”. I’ll wager that if you ask five pilots to define the “average pilot” you might get five different answers. Do you think a manufacture’s test pilot who determines that points along the curve can or cannot be accomplished qualifies as an average pilot? Most will agree they are at least above average pilots. So now we have an above average pilot who must then make a subjective determination whether an average pilot could successfully complete a point on the H-V curve. Do you also think, in making this determination, the manufacture’s test pilot just might be influenced by that rectangular piece of paper he gets from his employer every two weeks? Sure, so the way it works is that the manufacture’s test pilot will demonstrate that points along the curve can be accomplished. Then an FAA test pilot will duplicate those points and the FAA will, in essence, determine if an average pilot could be successful. Now, as far as how the word “successfully” is used. If you asked those same five pilots what they would consider a successful landing if their engine failed, again, I’ll bet you would get a few different responses. Some pilots just want to walk away, others would be satisfied with being able to crawl away, while others don’t want to bend or break a thing. As far as H-V testing is concerned, it’s clear. After the helicopter comes to a complete stop and all the dust has settled if there is any component that is not airworthy the landing is not successful. Beauty is not a consideration. The autorotation does not have to be pretty to be successful. In a number of H-V tests conducted at Robinson points inside the shaded area were successfully accomplished but it was felt they required too high a degree of pilot proficiency for the average pilot. After completing a sea level H-V test at maximum gross weight, it’s up to 7000 feet where the manufacturer can conduct the test at a lower weight but it must be at least OGE hover weight. For a more detailed discussion of the H-V diagram see the “Height–Velocity Diagram” essay.
ADDITIONAL TESTS
Engine and main rotor gear box cooling tests are required. Thermocouples are attached and all temperatures are measured. Then all measurements are corrected for a “hot day” which is 100ºF for piston engines. For turbine engines the temperatures the cooling measurements are corrected to become a flight manual limitation and is usually higher than 100ºF to allow for operation in very hot areas, for example, the R66 limitation is 122ºF (50ºC).
A 100 hour ground endurance test is also performed. The helicopter is anchored to the ground and run at high power for 100 continuous hours. During the test, 500 cyclic and pedal control reversals as well as 200 cycles from high to low power are made. One control reversal consists of moving the control from neutral to one stop then to the opposite stop and back to neutral. Since the helicopter is anchored to the ground, the loads induced are quite high (Some relief is given on the stop-to-stop requirement if the loads would exceed the maximum flight loads obtained in the strain survey). After the 100 hours the helicopter is completely disassembled and inspected. All the drive system and control mechanisms must still be serviceable.
Finally, a Function & Reliability test is completed by the FAA. The new helicopter model is typically flown for 300 hours by a number of different FAA pilots. These pilots assess the general flying and handling qualities of the aircraft and insure there are no recurring maintenance items. Even if the aircraft flies several hours per day, Function and Reliability tests may take several months.
THE AIRCRAFT TYPE CERTIFICATE
I think all will agree, it’s a long, hard and, in some cases, risky process to go from a single prototype with no flight hours to an FAA type certificate. The test program is fraught with weather delays, test results requiring design changes and numerous unexpected problems which make predicting the length of the process nothing more than a guess. Not only is it impossible to predict what problems will be encountered but, more importantly, how long it will take to solve those problems. Once the type certificate is issued, the manufacturer must demonstrate to the FAA that they can build aircraft to the same design specifications as the prototype and this is called the “production certification”. Interestingly, as you might expect with a company that had never produced aircraft previously, it took Robinson six months (March–October 1979) to go from the type certificate to the production certificate with the R22, whereas with the R66 the two certificates were awarded the same day.
Tim Tucker
October 2019